Cyclone engineers are using big data to make big improvements to road safety and traffic management. Anuj Sharma, associate professor of civil, construction and environmental engineering, and a team of researchers are using continuous traffic data streams – video, traffic volume, speed, backups, weather and more – to build automated, real-time traffic management tools. With support … Continue reading Data Driven: Smart Systems For Traffic Management
Category: Research Highlights
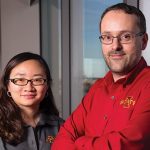
New Nylon, New Possibilities
Cyclone engineers Zengyi Shao and Jean-Philippe Tessonnier created a new type of biobased nylon that outperforms nylon created from petroleum chemicals. Shao and Tessonnier, both assistant professors of chemical and biological engineering, combined their expertise in biocatalysis and chemical catalysis to design a hybrid biomass-to-nylon process that integrates fermentation and downstream upgrading. This hybrid process … Continue reading New Nylon, New Possibilities
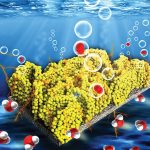
Bubbling With Renewable Fuel: Nanoscale Catalyst Splits Hydrogen From Water Quickly and Efficiently
At the macroscale, rust is a common, everyday material. But at the nanoscale, it might hold the promise of sustainable clean energy. Shan Hu, an assistant professor of mechanical engineering, is developing new catalysts made of rust nanostructures that convert light into fuel faster and cheaper than other leading catalysts. “Rust, or iron oxide, is … Continue reading Bubbling With Renewable Fuel: Nanoscale Catalyst Splits Hydrogen From Water Quickly and Efficiently
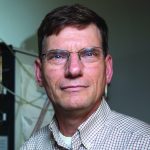
Jump-starting battery advancements
Solid electrolytes are key to making batteries more powerful and safer For more than 30 years, Steve Martin has been studying and characterizing different materials to identify properties that would allow for optimal energy transfer and storage in batteries. He says ceramic-like sulfide glasses may hold the solution, and now he’s working on a project … Continue reading Jump-starting battery advancements
FactBoard: Visualizing Data
Creating a real-time data-driven visual decision support system for the factory floor Guiping Hu has set out to make manufacturing production more efficient. The associate professor of industrial and manufacturing systems engineering is working on a project for the Digital Manufacturing and Design Innovation Institute (DMDII) to develop a shop floor decision support system called … Continue reading FactBoard: Visualizing Data
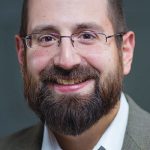
Ecosystems of support
Promoting an accessible, responsive approach to engineering education A new initiative in the Department of Electrical and Computer Engineering will help a pool of talented students pursue a degree in engineering. The project, called ECSEL: Electrical, Computer, and Software Engineers as Leaders, is part of the National Science Foundation’s Scholarships in Science, Technology, Engineering, and … Continue reading Ecosystems of support
Characterizing antimicrobial resistance
Interdisciplinary team uses systems approach to sequence microbial genes Historically, treatments for disease-causing microorganisms have relied heavily on the use of antimicrobial drugs. Adina Howe says this very practice (both when it’s used properly and when it’s misused), along with naturally occurring phenomena, has accelerated how quickly microorganisms are evolving into resistant strains. “If we … Continue reading Characterizing antimicrobial resistance
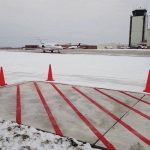
Safer, more sustainable aviation
Iowa State’s partnership in FAA program advances airport runways, operating technology Engineers from Iowa State are part of a collaborative partnership to help navigate and improve the complex, ever-changing aviation industry with innovative ideas and new research projects. “When you look at addressing any problem in the field, from the weather to security to operational … Continue reading Safer, more sustainable aviation
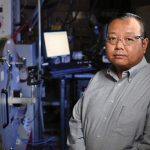
Increasing electric steel performance
Materials research will make electric motors more efficient, cost effective Can electric steel, a popular material that’s already a key functional material for modern society, get better? Jun Cui, an associate professor of materials science and engineering and a senior scientist at the U.S. Department of Energy Ames Laboratory, says it can. That’s why he’s … Continue reading Increasing electric steel performance
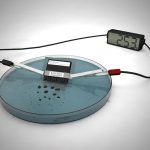
Self-destructing batteries
A practical solution for powering transient electronics Researchers at Iowa State have made significant progress in an effort to make transient electronic devices completely autonomous. Reza Montazami, an assistant professor of mechanical engineering and an associate of the U.S. Department of Energy’s Ames Laboratory, is leading a team that has developed a transient lithium-ion (Li-ion) … Continue reading Self-destructing batteries
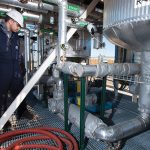
The road to biorenewable asphalt
Researchers scale-up plans to use soybean oil to produce bio-polymers A new Bio-Polymer Processing Facility located at Iowa State University’s BioCentury Research Farm gives researchers a broader understanding of what it would take to commercialize bio-polymers so they can be added to materials like asphalt. Eric Cochran, associate professor of chemical and biological engineering, and … Continue reading The road to biorenewable asphalt
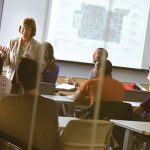
A new model of engineering education
Collaborative course design will transform education and develop the next generation of engineers Electrical and computer engineering technologies have evolved from simple electronics and computing devices to complex systems that profoundly change the world in which we live. Designing these complex systems requires a new way of thinking, including developing social, professional and ethical responsibility. … Continue reading A new model of engineering education
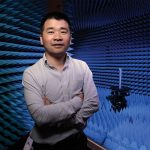
Making progress toward invisibility cloaks
Meta-skin suppresses scattering microwaves, hides objects from radar detection Two professors in electrical and computer engineering have made significant headway in an innovative stealth technology. Liang Dong, an associate professor who researches micro-nanofabrication, liquids and polymers, and Jiming Song, a professor who studies electromagnetics, have combined their areas of expertise to create a flexible, stretchable … Continue reading Making progress toward invisibility cloaks
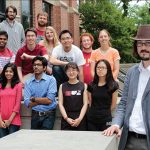
Nanostructures and Lego® Bricks
Advancing technology through practical and radical materials science Materials science, chemistry, physics and life. These are the driving forces behind the research in Ludovico Cademartiri’s laboratory at Iowa State. Cademartiri, assistant professor of materials science and engineering and associate scientist with the U.S. Department of Energy Ames Laboratory, says he and his graduate students work … Continue reading Nanostructures and Lego® Bricks
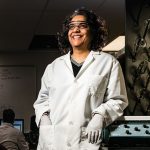
Engineering better health
Polymeric biomaterials combined with nanoscale delivery devices can improve preventative medicine Surya Mallapragada knows the value an engineering perspective can add to advancing the biomedical field. For years, Mallapragada, the Carol Vohs Johnson Chair in Chemical and Biological Engineering, has been researching the best ways to synthesize copolymers and nanoscale delivery devices to treat illnesses. … Continue reading Engineering better health
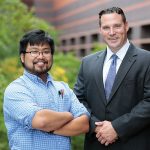
ATHENA Lab augments human capabilities
Engineering ideas and technologies enhance human performance, preserve safety and quality of life Developing telerobotics control systems, applying biomechanics for improved sports performance, creating visualization tools that improve battle space awareness. These projects and more are going on within the Augmentation and Training of Humans with Engineering in North America Lab. The lab, known as … Continue reading ATHENA Lab augments human capabilities
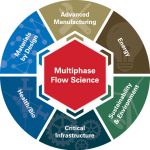
Leadership in multiphase flow discovery, education and practice
A cohesive group of researchers at Iowa State has joined together to accelerate discoveries in multiphase flow science and their transfer to industry. The group known as CoMFRE initially started out in 2002 as an informal way for faculty to share insights about related research projects. Now, the team is more formally organized with a … Continue reading Leadership in multiphase flow discovery, education and practice
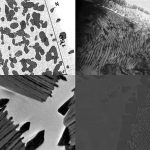
New heights for materials science
With his plans to develop an open-source manufacturing platform, Peter Collins is determined to make additive manufacturing a mainstream technology. Collins, who is an associate professor in materials science and engineering, says the advantages of additive manufacturing—using fewer resources, improving material performance and creating one-of-a-kind products—can be a game changer for manufacturers of all sizes. … Continue reading New heights for materials science
More data, more vulnerabilities
Protecting and securing data takes on many forms at Iowa State, where researchers in electrical and computer engineering are tackling big threats in the digital world: detecting malware on apps, improving online privacy and eliminating insider threats. Mathematical abstraction and software reasoning Suraj Kothari, Richardson Professor of Electrical and Computer Engineering, mixes theoretical ideas and … Continue reading More data, more vulnerabilities
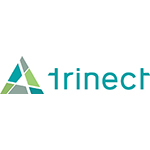
Engineers, elementary educators and future teachers partner to teach STEM
At Iowa State, integrating engineering into K-12 classrooms is a collaborative effort that spans across campus and into surrounding school districts. Trinect, a program funded by the National Science Foundation STEM-C Partnerships, brings together three groups to introduce engineering concepts to young students: engineering graduate students, preservice teacher students and cooperating elementary teachers from Des … Continue reading Engineers, elementary educators and future teachers partner to teach STEM
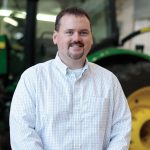
Market-ready solutions
Advanced ag machinery technology developed at ISU transfers to industry Matthew Darr’s research group strives to make an impact that extends knowledge and scientific development to the marketplace. “Seeing our results ported out to the public sector where we’re able to help agricultural producers with intelligent technologies — that’s our ‘why,’” he says. Darr, an … Continue reading Market-ready solutions
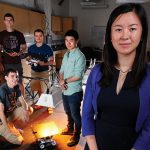
Power management in smart robots
Designing solar-powered vehicles for long-duration, high-efficiency missions Using renewable energy to power aerial and ground vehicles could change the way we handle aspects of environmental monitoring, search and rescue missions, surveillance, and agricultural practices. To navigate these sorts of dynamic environments, Ran Dai, an assistant professor in aerospace engineering and Black and Veatch Faculty Fellow, … Continue reading Power management in smart robots
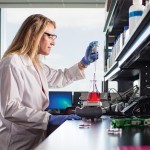
Soil and water samples illuminate antibiotic resistance
Studies of manure application reveal antibiotic resistance movement While using manure as an organic source of fertilizer has helped the agricultural industry maintain balance in integrated crop and livestock systems, there is some risk the manure contains bacteria that may contaminate water bodies if transported off the field by rain. Michelle Soupir, an associate professor … Continue reading Soil and water samples illuminate antibiotic resistance
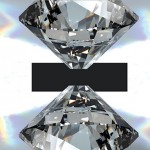
Materials under extreme conditions
Search for new materials and phases under high pressure and large plastic shear When you look at the properties and strength of a diamond, which is created under intense heating and pressure, it’s clear there’s value in understanding how it’s synthesized. Phase transformations under high pressure, like when graphite is formed into a diamond, are … Continue reading Materials under extreme conditions
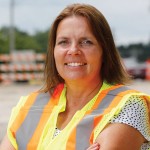
Easing traffic headaches
InTrans performs need-based research to improve transportation infrastructure Since Iowa State’s Institute for Transportation (InTrans) first began as the Local Transportation Information Center in 1983, researchers have transformed the surface transportation landscape through innovative methods, materials and technologies. Now, with specialization in areas that range from work zone safety and traffic engineering to sustainable pavement … Continue reading Easing traffic headaches
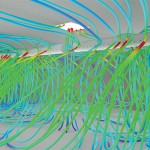
A faster way to optimal solutions
Using simulation and modeling to improve sustainable buildings Insurmountable data is no match for Baskar Ganapathysubramanian. In fact, he says the more data, the better. That way he’s sure to evaluate every possible scenario within the models he creates. Ganapathysubramanian, associate professor of mechanical engineering, uses mathematical techniques and computational tools to solve a variety … Continue reading A faster way to optimal solutions
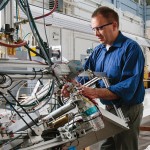
Customized products, processes
Delivering advanced manufacturing technology ready for commercialization Researchers in Iowa State’s industrial and manufacturing systems engineering (IMSE) department are major players in the country’s goal to strengthen the resurgence of manufacturing in America. With their projects to be supported by three of the recent federal manufacturing research centers – America Makes, the Institute for Advanced … Continue reading Customized products, processes
Wind characterization leads to new innovations
Expanding wind energy volume takes an interdisciplinary perspective Aerospace engineering and atmospheric sciences are joining forces and technology at Iowa State to better understand airflow and wind shear on wind farms. Using computer models, wind and icing tunnel experiments, and field measurements, the researchers say their work can depict individual turbine dynamics, turbine-to-turbine interaction and … Continue reading Wind characterization leads to new innovations
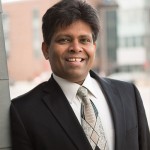
Taking cyberinnovation to the farm
Data from soil sensors gives researchers and farmers insight into sustainable agricultural practices Maintaining soil health by protecting land suitable for growing crops continues to be a priority as the world’s population rises. Issues like managing the nitrogen cycle, which is also one of the 14 Grand Challenges identified by the National Academy of Engineering, … Continue reading Taking cyberinnovation to the farm
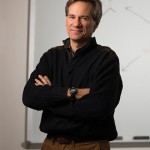
Shifting the paradigm of biorenewable chemicals
CBiRC focuses on platform technologies and new chemicals from biomass feedstocks to replace petrochemicals When the National Science Foundation Engineering Research Center for Biorenewable Chemicals (CBiRC) was founded in 2008, its approach of combining biology and chemistry to develop sustainable biobased chemicals was a novel idea. “At the time, we were trying decide if there … Continue reading Shifting the paradigm of biorenewable chemicals