Doug Jacobson’s leadership in cyber security research and education began before high-speed internet, smartphones and Google. It even predates the term “cyber security” itself. Jacobson, Stanley Chair in Interdisciplinary Engineering, University Professor of electrical and computer engineering, and director of the Center for Cybersecurity Innovation and Outreach, was one of the few who saw “information … Continue reading Writing the Script
Category: Research Highlights
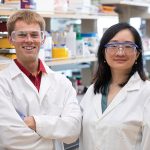
Powerhouse of the cell, key to new treatments: Mitochondrial DNA manipulation offers insights into related diseases
If you remember just one thing from high-school biology, it’s that the mitochondria are the powerhouses of the cell. But could mitochondria also hold the key to gene therapy and new tailored drug development for diseases and disorders? Zengyi Shao, Vernon Guse Faculty Fellow and associate professor of chemical and biological engineering, and her team … Continue reading Powerhouse of the cell, key to new treatments: Mitochondrial DNA manipulation offers insights into related diseases
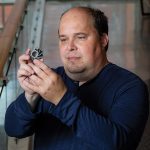
More than the sum of its parts
Additive manufacturing (AM) holds the promise of transforming nearly every way that materials and components are conceived, designed, produced and used in service. The possibilities and expected innovations are numerous, and their realization requires a truly multidisciplinary cadre of researchers. Here at Iowa State, researchers are at the forefront of additive manufacturing, conducting leading research … Continue reading More than the sum of its parts
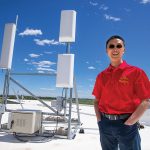
Wireless Living Lab: At-scale, real-world broadband testbed for connected rural communities
Iowa State is building a wireless “living lab” in central Iowa, laying the foundation for more affordable rural broadband service. The project will create a research testbed for a wide range of wireless technologies across Iowa State’s campus, the city of Ames and surrounding farms and rural communities in central Iowa – with an application … Continue reading Wireless Living Lab: At-scale, real-world broadband testbed for connected rural communities
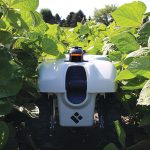
A.I. for Breakthrough Innovations
NSF AI Research Institute: Using digital twins to multiply crop production A new $20 million National AI Research Institute led by Iowa State will use the latest artificial intelligence tools to develop digital twins of individual crop plants and entire farm fields. The discoveries will help plant breeders improve crop varieties and farmers boost production. … Continue reading A.I. for Breakthrough Innovations
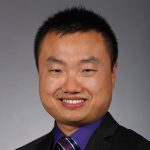
Tapping smart meters’ potential for grid resilience
Problem: Enhanced electric grid monitoring is needed to promote renewable integration while ensuring reliability, but current approaches rely on expensive sensors. Smart meters that electric utilities are already using for billing have potential, but without new computing innovations, smart meters only can provide limited insights into grid performance. Project: Wang will unlock the potential of … Continue reading Tapping smart meters’ potential for grid resilience
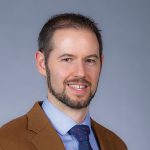
Biomanufacturing better treatments
Problem: Therapeutic cells show promise as a new treatment option for chronic illness, but advances must be made in manufacturing reproducibility to get therapeutic cells out of clinical trials and into widespread use. Project: Reuel will develop novel, real-time sensors and reinforcement learned (RL), dynamic control policies to improve reproducibility in large-scale manufacturing of differentiated, … Continue reading Biomanufacturing better treatments
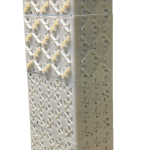
Smorphacade
“The Smorphacade project is the first-of-its-kind collaboration between structural engineers, wind engineers and control engineers to turn a passive building façade into a live façade that protects the building against wind load and turbulence,” said Alice Alipour, associate professor of civil, construction and environmental engineering. Alipour leads a team that’s combining a network of pressure, velocity and acceleration sensors strategically positioned on building surfaces. When excessive vibration-causing flow conditions are detected, the Smorphacade will change surface roughness or smoothness to mitigate inter-story building movements.
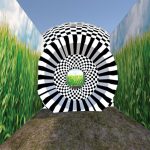
Virtual Reality, Real Cybersickness
Cybersickness. No, it’s not being tired of the constant stream of new emails in your inbox. It’s the physical illness some people feel in virtual reality environments. Angelica Jasper, a Ph.D. student in Humana Computer Interaction, is studying how to prevent VR users from experiencing nausea, disorientation, and oculomotor strain.
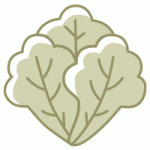
Engineering sustainable, safe food from the field to the table
A team led by Hongwei Zhang, professor of electrical and computer engineering, has developed CyNet, an open-source, wireless networking infrastructure to support data-driven research. CyNet takes on the challenge of quickly and reliably transmitting agricultural measurements from the crop field to plant scientists’ computers – and serves as a prototype for bringing much-needed broadband to rural areas. Food safety testing usually takes place in the lab by highly training technicians, but on-site, real-time, low-cost monitoring is our best tool to stop contaminated food from reaching consumers. Carmen Gomes, associate professor of mechanical engineering, is making key innovations in biosensor fabrication and system design to ensure food safety.
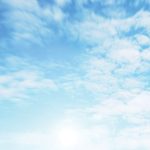
Boeing Undergraduate Research Fellowship program takes flight
A new partnership with Boeing pairs undergraduate students with College of Engineering faculty and Boeing engineers to get hands-on research experience in cutting-edge projects in aerospace engineering, cyber security, autonomous systems, machine learning and more. “The Boeing Undergraduate Research Fellowship has created opportunities for undergrads to learn research by doing, work on high-impact projects, and get familiar with the intellectual property considerations of industry-backed research,” said Arun Somani, associate dean for research, Anson Marston Distinguished Professor in Engineering, and Philip and Virginia Sproul Professor in Electrical and Computer Engineering.
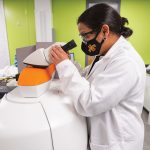
Nanostars guide the way to better disease treatment and diagnosis
Rizia Bardhan, associate professor of chemical and biological engineering and Iowa State University Nanovaccine Institute researcher, is making life-saving advances in disease diagnosis and treatment by integrating nanoparticles and Raman spectroscopy.
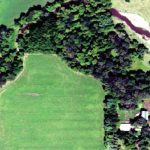
All about (data) connections: Precision conservation at the FEW nexus
Amy Kaleita, professor of agricultural and biosystems engineering, works at the intersection of food, energy, water and land use systems on data-driven precision conservation. Where there’s good soil and water data, she uses it, where there’s gaps in the data, she creates new models to estimate variables, and where there’s neither existing data nor a good model, she designs new sensing systems. And in all cases, Kaleita says she focuses on the connections – between complex natural systems, land uses and more – to protect our land and water.
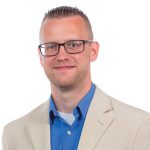
Beyond batteries: New energy systems for a sustainable future
Incremental improvement is the goal of most battery research programs, but Cary Pint isn’t looking to build on existing ways of doing things. He’s out to create new systems of energy storage for a sustainable future. “My background brings together diverse experiences in both academia and industry and across many different disciplines. That allows me to approach these problems in new and unique ways – and I’ve built a multidisciplinary research team that does the same,” said Pint, Charles Schafer (Battelle) Chair in Engineering and associate professor of mechanical engineering.
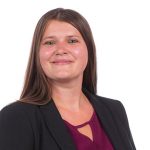
In this together: Collaborative networks create community resilience
Cyclone Engineer Cristina Poleacovschi studies how to build community resilience during challenges like natural disasters or economic shocks. Poleacovschi’s research suggests steps to increase shared resilience – even in unprecedented community challenges like the COVID-19 pandemic:
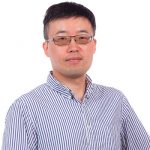
Storage systems keeping data safe
Storage systems are the fundamental computing building block of our modern lives – and like physical building blocks, computer storage systems must function well in fair weather as well as sudden disturbances to keep data safe.
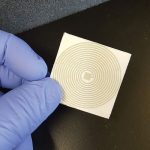
Sensors for closed systems open up possibilities in biotech
Ask Nigel Reuel about his research and you start to hear a pattern in how he describes his goals: “merging,” “collaborating,” “tying together,” “multidisciplinary.” Look at his career, and you see how he’s combined industry experience with in-the-lab creativity to create engineering innovations. “When I was at DuPont, one of my roles was a technology scout, looking around for the best ideas that would be useful for core businesses, and I started to see that many other companies were looking to university professors as well to develop the next wave of innovation,” said Reuel, assistant professor of chemical and biological engineering. “I realized where I could really push innovation and bring together teams of strong players would be joining academia.” Since coming to Iowa State, Reuel has succeeded in becoming the “entrepreneurial professor” he set out to be.
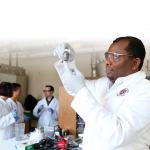
Engineering innovations for a better world
Iowa State University is once again listed among the top 100 worldwide universities granted U.S. patents during a calendar year. Iowa State has made the list every year since 2013 when the rankings began.
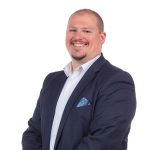
Engineering probiotics to fight illness
Our gut microbiome – a collection of complex and varied microbes – is important to our health far beyond our digestive tract. We know that too much “bad” bacteria can make us ill, but the opposite may also be true. Engineering “good” bacteria could be key to fighting illness. Mansell seeks to overcome one big challenge of introducing new good bacteria into the gut environment: When we eat foods rich in live cultures or take a supplement that introduces probiotics into our gut, then the probiotics themselves need something to feast on if they are to multiply and remain in place.
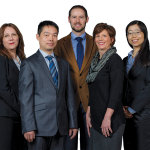
Filling the GAPS
Could graduate students build more than just research skills during their thesis projects? An interdisciplinary project led by Cyclone Engineer Shan Jiang aims to address this question through the first of its kind Graduates for Advancing Professional Skills (GAPS) program.
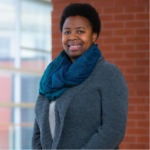
New Materials for Nuclear Realtors
Trishelle Copeland-Johnson is a Ph.D. student in materials science and engineering. She was recently awarded an American Society for Nondestructive Testing Fellowship for her research in new materials for nuclear reactors.
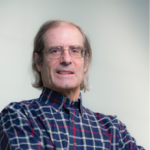
Expect Turbulence
Paul Durbin is creating new hybrid, adaptable computational models to predict transitions from laminar fluid flow to turbulence and to simulate the turbulent flow. Right now, engineers rely on two types of turbulence modeling: average flow calculations, which offer high efficiency, but less detail, and eddy-resolving calculations, which offer more detail about turbulence, but take more time and are often cost prohibitive.
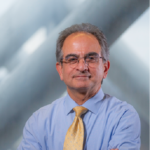
Nondestructive Direction – New Center Director, Continued National Leadership
Reza Zoughi, a research and teaching leader in nondestructive evaluation, has joined Iowa State University as the director of the Center for Nondestructive Evaluation and the Kirby Gray (Battelle) Chair in Engineering in the Department of Electrical and Computer Engineering.
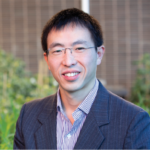
Growing Collaboration: Optimization and machine learning meet plant genomics
Lizhi Wang, associate professor of industrial and manufacturing systems engineering, is applying process optimization and machine learning to improve crops’ adaptability to new environmental conditions. Wang, also an Iowa State Plant Sciences Institute Faculty Scholar, serves as the principal investigator for the project supported by a $2 million grant from the National Science Foundation.
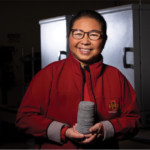
3-D Printing Concrete Rapid Solutions with a Steady Material
If 3-D printing can quickly and efficiently create machine parts, could it also quickly and efficiently create bridges and buildings after natural disasters? Kejin Wang, Wilson Professor of Engineering in civil, construction and environmental engineering, is leading advanced research in the emerging field of 3-D concrete printing. She aims to add 3-D concrete printing to civil and construction engineers’ toolbox.
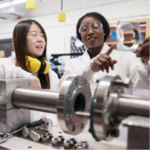
Illuminating Invisible Injuries With High-Impact Research
The devastating effects of traumatic brain injuries caused by flying, penetrating objects following a blast are well documented. What remains unknown are the specifics of how shock waves, created immediately after detonation of explosive devices, damage the brain.
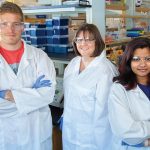
Tiny components, big accomplishments for Nanovaccine Institute’s young scientists
The headlines and feature stories have become frequent for the Iowa State University-based Nanovaccine Institute, a multidisciplinary network of scientists devoted to transforming the development and delivery of vaccines and therapeutics that will fight deadly diseases worldwide. Led by Iowa State Chemical and Biological Engineering Anson Marston Distinguished Professor and Vlasta Klima Balloun Faculty Chair … Continue reading Tiny components, big accomplishments for Nanovaccine Institute’s young scientists
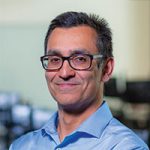
Raj Raman
Raj Raman, Morrill Professor of agricultural and biosystems engineering, sees the purpose of any university as creating new knowledge and building human capital. In his research to improve engineering education, Raman does both.
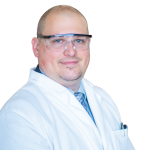
Engineering health at the breakfast table
When mom said “breakfast is the most important meal of the day,” she couldn’t have imagined what Cyclone Engineer Tom Mansell has cooking. Mansell is creating new probiotics and prebiotics that will treat disease as you eat your morning cup of yogurt.